Have you ever wondered how light can knock electrons out of a material? This fascinating process is called the photoelectric effect. It’s not just a cool science experiment—it’s a cornerstone of modern physics. Back in 1905, Albert Einstein explained that light isn’t just a wave; it’s made of tiny energy packets called photons. This discovery changed everything. It showed why only certain frequencies of light can eject electrons, no matter how bright the light is. Also, Einstein’s work even earned him a Nobel Prize in 1921! Today, the photoelectric effect powers technologies like solar panels and light sensors, proving how a simple idea can revolutionize the world.
Principles of the Photoelectric Effect
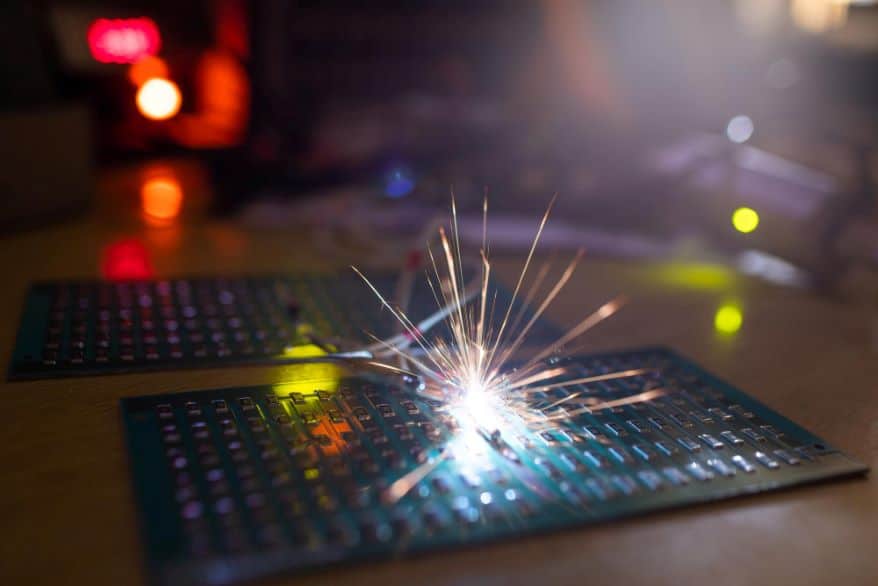
Understanding the photoelectric effect starts with grasping its core principles. Let’s break it down step by step.
The Role of Photons in the Photoelectric Effect
You’ve probably heard of photons—they’re the tiny packets of energy that make up light. When photons hit a material, they transfer their energy to the material’s electrons. If the energy of a photon is high enough, it can knock an electron out of the material. This is the essence of the photoelectric effect.
Scientists like Heinrich Hertz and Philipp Lenard helped prove this. Hertz discovered that ultraviolet light could create an electric current in metals. Lenard took it further, showing that the frequency of light—not its intensity—determines whether electrons are ejected. These experiments confirmed that photons interact with electrons in a way that matches the predictions of quantum mechanics.
Threshold Energy and Quantum Nature of Light
Here’s where the quantum nature of light comes into play. Each photon carries energy proportional to its frequency, calculated as E = hf (where h is Planck’s constant and f is the frequency). For an electron to escape a material, the photon’s energy must exceed the material’s work function—a kind of energy barrier. If the frequency of the light is too low, no electrons are emitted, no matter how bright the light is. This threshold frequency is a direct result of light’s quantum behavior.
Key Characteristics of the Photoelectric Effect
The photoelectric effect has some fascinating traits. First, the process happens instantly—there’s no lag time between the light hitting the material and the electrons being ejected. Second, the kinetic energy of the emitted electrons depends on the light’s frequency, not its intensity. Consequently, third, each material has its own unique threshold frequency. These characteristics have been verified through countless experiments and are key to understanding how the photoelectric effect works.
Conditions for the Photoelectric Effect
What makes the photoelectric effect happen? It’s not just about shining light on a material. Therefore, certain conditions must be met for the release of electrons to occur. Let’s explore these key factors.
Threshold Frequency and Work Function
For the photoelectric effect to take place, the incident light must meet a minimum condition for the photoelectric effect: its frequency must be high enough. This is called the threshold frequency. If the light’s frequency is too low, even the brightest beam won’t eject electrons. Why? Because the energy of the photons depends on their frequency, as described by the equation E = hf.
The work function of a material also plays a big role. This is the minimum energy needed to knock an electron loose. Materials with a higher work function require photons with more energy (and thus higher frequency) to cause the emission of electrons. For example, cesium, with a work function of 2.1 eV, needs less energetic photons compared to gold, which has a work function of 5.1 eV.
Here is a table for the work function of some metals:
Material | Work Function (eV) |
Cesium | 2.1 |
Potassium | 2.3 |
Sodium | 2.28 |
Magnesium | 3.68 |
Aluminum | 4.08 |
Gold | 5.1 |
Material Properties and Their Influence
Not all materials respond to light the same way. Metals like cesium and potassium, with low work functions, are more likely to emit photoelectrons when exposed to light. These materials are often used in devices like solar panels because they require less energy to eject electrons. On the other hand, materials with higher work functions, like gold, need more energetic photons, making them less efficient for photoelectric applications.
Absence of Lag Time
Here’s something fascinating: the photoelectric effect happens instantly. In fact, the moment the incident light hits the material and meets the minimum condition for the photoelectric effect, electrons escape from it. Particularly, there’s no delay. This shows that the energy transfer from photons to electrons is immediate, a key feature that supports the quantum nature of light.
Intensity Independence
You might think brighter light would mean more energetic electrons, but that’s not the case. The energy of the ejected electrons depends only on the frequency of the light, not its intensity. Brighter light does increase the number of photoelectrons emitted, but their energy remains unchanged. This is why the photoelectric effect is so unique—it’s all about the quality (frequency) of the light, not the quantity.
Mathematical Explanation of the Photoelectric Effect
Understanding the math behind the photoelectric effect might sound intimidating, but it’s actually pretty straightforward. Let’s break it down step by step.
Einstein’s Photoelectric Equation
Einstein’s photoelectric equation is the key to understanding how light interacts with matter. It’s written as:
1/2 m v²max = hν – φ₀
Here’s what this means:
- The left side, 1/2 m v²max, represents the maximum kinetic energy of the ejected electrons.
The right side, hν, is the energy of the incoming photon, where h is Planck’s constant and ν is the frequency of the light.
- Furthermore, the term φ₀ is the work function, which is the minimum energy needed to free an electron from the material.
This equation shows that the maximum kinetic energy of the photoelectrons depends on the frequency of the light and the material’s work function. If the light’s frequency is below a certain threshold, no electrons are emitted, no matter how intense the light is.
Photon Energy and Electron Kinetic Energy
When a photon hits a material, it transfers its energy to an electron. This energy is having two parts:
The energy needed to overcome the work function (binding energy).
The leftover energy, which becomes the electron’s kinetic energy.
You can express this relationship mathematically as:
KE_e = hf – BE
Here, ( KE_e ) is the maximum kinetic energy of the ejected electron, ( hf ) is the photon’s energy, and consequently, ( BE ) is the binding energy (or work function).
Think of it like this: the photon gives all its energy to the electron. Moreover, part of it helps the electron escape the material, and the rest determines how fast the electron moves after it’s free.
These equations explain why the photoelectric effect depends on the frequency of light, not its intensity. They also highlight the quantum nature of light, which behaves as both a wave and a particle.
Applications of the Photoelectric Effect
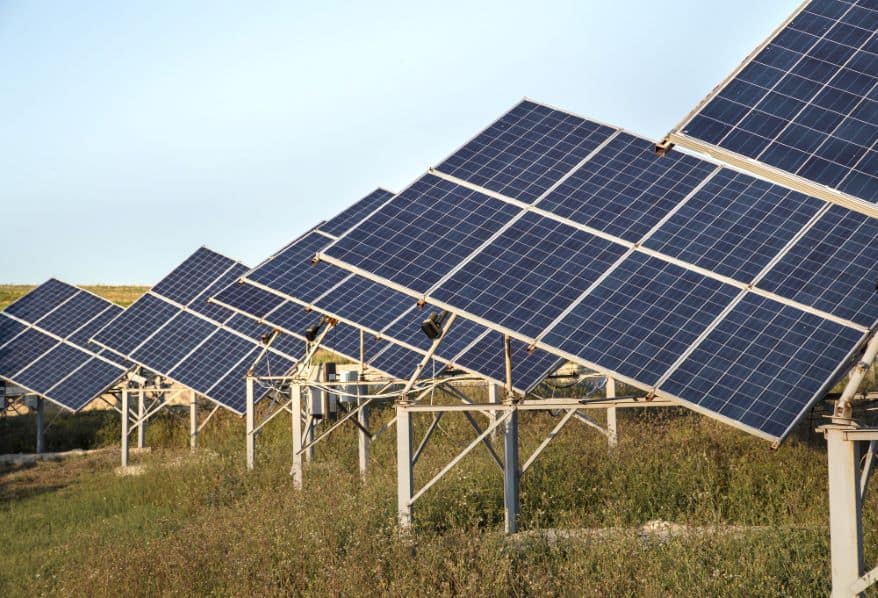
The photoelectric effect isn’t just a fascinating scientific concept—it’s the backbone of many technologies you use or encounter daily. Let’s dive into two of its most impactful applications.
Solar Panels and Renewable Energy
Have you ever wondered how solar panels turn sunlight into electricity? It’s all thanks to the photoelectric effect. Here’s how it works:
When sunlight hits the surface of photovoltaic cells, photons excite electrons in the semiconductor material.
This excitement gives electrons enough energy to break free from their atomic bonds.
- Further, these free electrons generate an electric current, which powers everything from homes to satellites.
Therefore, without the photoelectric effect, converting light energy into electricity wouldn’t be possible. In fact, this progress has been a game-changer for renewable energy, helping reduce reliance on fossil fuels. Every time you see a solar panel, you’re witnessing the photoelectric effect in action.
Fun Fact: The efficiency of modern photovoltaic cells has doubled in the last two decades, making solar energy one of the fastest-growing renewable energy sources.
Light Sensors and Photodetectors
Light sensors and photodetectors also rely on the photoelectric effect to function. These devices convert light into electrical signals, enabling a wide range of applications. Here’s what happens:
When photons hit a photodetector, they create electron-hole pairs in the semiconductor material.
- This process generates an electrical signal, which can detect light or measure its intensity.
Photodetectors are everywhere. They’re in safety light curtains that protect workers in factories. Also, they’re in smoke detectors that alert you to fires. Moreover, X-ray machines and pulse oximeters use the photoelectric effect to save lives. Moreover, these devices showcase how the photoelectric effect makes modern technology smarter and safer.
Tip: Next time you use a remote control or walk through an automatic door, remember that the photoelectric effect is quietly at work behind the scenes.
The photoelectric effect isn’t just a scientific concept—it’s a game-changer. It revealed light’s dual nature, laying the foundation for quantum mechanics. Additionally, you’ve seen how it powers solar panels and drives innovation in sensors. By understanding photons and their energy, you’re exploring the very principles that shape modern technology and sustainable solutions.
References:
Niaz, M., Klassen, S., McMillan, B., & Metz, D. (2010). Reconstruction of the history of the photoelectric effect and its implications for general physics textbooks. Science Education, 94(5), 903–931. https://doi.org/10.1002/sce.20389
FAQ
What is the particle nature of light, and how does it relate to the photoelectric effect?
The particle nature of light means light behaves like tiny energy packets called photons. Further, these photons transfer energy to an electron, causing it to escape the material.
Why does the photoelectric effect depend on light frequency, not intensity?
The energy of a photon depends on its frequency. If the frequency is too low, the photon can’t eject an electron, no matter how bright the light is.
How does the photoelectric effect prove quantum mechanics?
The photoelectric effect shows light behaves as particles (photons) and follows quantum rules. Also, it explains why only certain frequencies can eject an electron, supporting quantum theory.
To stay updated with the latest developments in STEM research, visit ENTECH Online. This is our digital magazine for science, technology, engineering, and mathematics. Furthermore, at ENTECH Online, you’ll find a wealth of information.
In addition, we offer insights and resources to fuel your curiosity. Ultimately, our goal is to inspire your passion for new scientific discoveries. Moreover, ENTECH Online provides everything a teen wants to know for career planning.